Tracing the History of Polymeric Materials: Acetal
The road from discovery in the lab to commercial viability can be long, and this was certainly the case for acetal polymers.
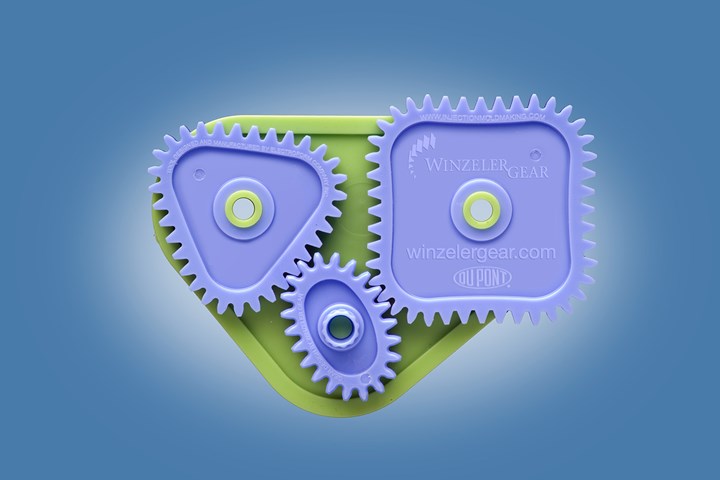
Acetals’ high crystallinity and mechanical properties, together with high dimensional stability and low coefficient of friction, have made them preferred materials for gears, bushings and wear strips. (Photo: Winzeler Gear)
The commercial introduction of the class of materials known as acetals (or polyoxymethylene, POM) occurred in 1960 when DuPont started producing the material known as Delrin. However, as we have seen many times during this series, the road from discovery in the lab to commercial viability can be long, and this was certainly the case for acetal polymers.
Hermann Staudinger studied the polymerization of formaldehyde in the early 1920s as part of his research into the structure of polymers. Staudinger advanced the theory that polymers derived their properties from the high molecular weight and extended chain shape of the individual molecules. At the time, this was heresy. Most prominent organic chemists at the time believed that polymers were colloidal aggregates of small molecules.
But Staudinger proposed that those structures could not account for the properties of the materials that were being discovered, and he was able to show that the individual acetal polymer chains were, in fact, larger than the crystals of which they were a part. This, of course, never happens in classical materials, where the building blocks that form the crystal are smaller than the crystal itself.
As useful as the discovery of polymerized formaldehyde was in making Staudinger’s case for our modern understanding of polymers, the material itself exhibited significant problems with thermal stability, tending to rapidly depolymerize and making melt processing impossible. In 1952, DuPont chemists synthesized a version of the material with the polymer chains terminated by a chemical group known as a hemiacetal. This was a prototype of what would become the commercial acetal homopolymer.
In 1956 DuPont filed for patent protection of this invention. However, the hemiacetal chain end also lacked the thermal stability needed for melt processing, and it was another DuPont chemist, Stephen Dal Nogare, who discovered that reacting the hemiacetal end group with acetic anhydride stabilized the polymer sufficiently to allow for melt processing. DuPont completed its first production plant for Delrin in Parkersburg, W.Va., in 1960 and a new polymer became available. In the same year, Celanese completed its research on an acetal copolymer that was introduced commercially in 1962 under the tradename Celcon, manufactured under a joint venture with Hoechst AG, with the initial plant located just outside Frankfurt, Germany. The Hoechst commercial offering, Hostaform, became available in the following year.
Processors familiar with acetal materials are well aware that process control with these resins can be challenging. The early materials provided a very narrow melt-processing temperature window and molders experienced significant problems with plateout. Over the six-decade history of these materials, the manufacturers have made continuous improvements in melt stability, and the materials produced today are much more user-friendly. Nevertheless, most of us have heard at least one story of a hopper being launched from its moorings or a plant being emptied of its employees due to the overheating of an acetal polymer and the consequent production of formaldehyde.
Processors familiar with acetal materials are well aware that process control with these resins can be challenging.
In addition, acetals have legendary sensitivity to anything containing a halogenated compound. Therefore, the processing literature warns against allowing the material to come in contact in the melt state with PVC. Other materials that can cause problems include melt-processable rubber compounds like Santoprene and Alcryn as well as materials that employ halogenated flame retardants.
Even with the advances in thermal stability that have occurred over the years, the rapid rate of depolymerization that occurs in acetals once decomposition begins makes the detection of degradation challenging. Analytical methods for detecting reductions in polymer chain length, such as melt flow rate, intrinsic viscosity, and gel-permeation chromatography, rely on the presence of a variety of reduced chain lengths in the degraded material. In the melt state, acetals depolymerize so efficiently that there is almost a complete absence of these reduced chains. Consequently, these conventional tests usually fail to detect degradation that can occur during processing. However, degradation that occurs in the solid state, such as in hot water, takes place more gradually and will register an increase in melt flow rate or a decrease in intrinsic viscosity.
Because the copolymer employs a number of ethylene-like segments within the chain, it enjoys a slightly greater degree of thermal stability than the homopolymer. This, coupled with the fact that the copolymer has a lower melting point, affords a wider processing window on both the low and high end of the allowable temperature range. This improved stability also carries over into the solid state, where acetal copolymer exhibits improved resistance to hot water, chlorine, and strong acids and bases.
However, the homopolymer has a higher degree of crystallinity. This translates into greater strength and stiffness and better resistance to creep and fatigue. While both copolymers and homopolymers exhibit a low coefficient of friction and excellent bearing properties, the higher crystallinity of the homopolymer produces a harder surface and superior tribological performance.
The history of the development of these materials over six decades is one of attempting to improve the thermal stability of the homopolymer to more closely approach the advantages of the copolymer, while the copolymer manufacturers have sought to improve on the mechanical strengths of their materials to bring them closer to the performance of the homopolymer. Neither side has succeeded completely in closing this gap, but the two material families are closer today than they were in their formative years.
As a polymer family, acetals extended the capabilities of their semi-crystalline predecessors: nylons and polyesters. Acetals possess a higher degree of crystallinity than either nylons or polyesters, giving them the superior mechanical properties that make them the preferred materials for gears, bushings, and wear strips. Nylons, in their dry-as-molded state, offer many of the same advantages. But these materials are substantially affected by their well-known tendency to absorb moisture, a problem that acetals do not display. Acetals’ high crystallinity also provides for superior solvent resistance. And while acetals do share with nylons and polyesters a susceptibility to hydrolysis, this process occurs more slowly in acetals, particularly in the copolymers. This accounts for the fact that while nylons and polyesters must be dried to particular moisture levels in order to be successfully processed, most grades of acetal can be molded without predrying.
Acetals exhibit some other important differences from nylons and polyesters. The melting point of acetal polymers is somewhat lower, 178 C (352 F) for the homopolymer and 165 C (329 F) for the copolymer. This limits the elevated temperature range at which the materials can be used, and when susceptibility to oxidation is factored in, long-term performance is limited to 90-100 C (194-212 F). The lower melting point is accompanied by a lower glass-transition temperature, well below room temperature. This is, in part, what allows for the achievement of the high degree of crystallinity. It also means that acetal resins can continue to crystallize at room temperature while nylons and polyesters do not.
Acetal resins can continue to crystallize at room temperature while nylons and polyesters do not.
This continued crystallization causes some dimensional changes that can go on for 24 to 48 hours after parts have been molded and may occur for a longer period of time if the material is not molded in a hot mold that allows for the achievement of optimal crystallization. The design guides written by the manufacturers of these materials place a great deal of emphasis on the importance of elevated mold temperature to ensure optimal part performance and long-term dimensional stability. The continued crystallization also means that mechanical properties will develop for several weeks after the parts have been molded. Some old design guides for Delrin have an interesting footnote at the bottom of the data sheet stating that properties were measured 21 days after specimens were molded. There is a reason for that.
Semi-crystalline polymers are typically lacking in ductility, and impact-modification strategies have been developed to improve their toughness by incorporating elastomeric materials. This has been done successfully, first in nylons and then later in polyesters, and these same improvements have been extended to acetals. The impact modifier of choice in acetals is usually polyurethane, which may introduce the need for drying the raw material.
Obtaining an optimal bond with glass fiber has proven to be more challenging in acetals than in nylons and polyesters. The problem was solved first in copolymers and good coupling of glass fiber to this polymer has been available for over 40 years. But in homopolymer it has only been in the last 15 years that a true glass-fiber reinforced option has been available. Prior to that time, glass fiber in homopolymer was a filler, not a reinforcement, due to the poor bonding of the glass fiber to the polymer.
Finally, to this point, acetals have not been made that can be sold as flame retardant. This is a matter of chemistry. Formaldehyde, the primary decomposition product from acetal polymers, is highly flammable and suppressing that would require massive amounts of an additive that would likely leave the material with a set of mechanical properties very different from those we have come to expect from this material family.
Despite all these caveats, acetals have advanced the use of plastics in ways that no other polymer could. Gears are a key example of this, and the design guides written around plastic gears have largely been inspired by the existence of acetal polymers. Without these materials many moving parts would still be made from metals. In addition, acetals are the binder of choice in metal injection molding.
In our next installment, we will look into a material that was introduced about 30 years ago, then disappeared from the marketplace, and now appears to be making a comeback.
ABOUT THE AUTHOR: Michael Sepe is an independent materials and processing consultant based in Sedona, Ariz., with clients throughout North America, Europe, and Asia. He has more than 45 years of experience in the plastics industry and assists clients with material selection, designing for manufacturability, process optimization, troubleshooting, and failure analysis. Contact: (928) 203-0408 • mike@thematerialanalyst.com
Related Content
Density & Molecular Weight in Polyethylene
This so-called 'commodity' material is actually quite complex, making selecting the right type a challenge.
Read MoreHow to Select the Right Tool Steel for Mold Cavities
With cavity steel or alloy selection there are many variables that can dictate the best option.
Read MoreThe Strain Rate Effect
The rate of loading for a plastic material is a key component of how we perceive its performance.
Read MoreHow to Set Barrel Zone Temps in Injection Molding
Start by picking a target melt temperature, and double-check data sheets for the resin supplier’s recommendations. Now for the rest...
Read MoreRead Next
Tracing the History of Polymeric Materials: Polyesters
Beyond PET, PBT and their analogues, development of polyester chemistry led to unsaturated thermosetting resins, copolyester thermoplastic elastomers, liquid-crystal polymers and, most recently, biopolymers.
Read MoreTracing the History of Polymeric Materials: The Differences Between Nylons & Polyesters
In many respects, nylons and polyesters appear to be interchangeable. But there are interesting differences in the properties of these two families that arise from their chemical structures.
Read MoreTracing the History of Polymeric Materials: PBT
The slow crystallization of PET polyester made it a poor option for processes like injection molding. This led to the development of more molder-friendly options such as PBT.
Read More