Materials: Annealing Tips for Semi-Crystalline Polymers: Part 4
You can forgo the elevated mold temperatures normally recommended for high-performance semi-crystalline materials. But it’s risky and likely to yield parts that under-perform expectations … assuming that they emerge from the annealing process looking anything like the drawing.
High-performance semi-crystalline polymers typically have high glass-transition temperatures. This results in the need for high mold temperatures to ensure that an appropriate level of crystallinity is established in the part. Polymers such as PPS, PEEK, PPA, SPS, and even some PET polyesters fall into this high-performance class where mold temperatures of at least 120 C (248 F) and as high as 200 C (392 F) are needed in order to achieve the desired structure. Some PET suppliers have developed nucleating technologies that allow for development of an appropriate level of crystallinity while still using mold temperatures that can be achieved with traditional water heating.
In the case of PPA, almost all suppliers of this class of materials have developed fast-crystallizing grades as an alternative to the original materials that required the more demanding mold temperatures. However, this improvement was achieved by manipulating the chemistry of the polymer so that the glass-transition temperature (Tg) is reduced. This is something that material suppliers neglect to mention, and while the molder may be very pleased to be able to produce parts without resorting to extreme mold temperatures, the end user may not be so enthusiastic.
The accompanying graph shows a plot of modulus as a function of temperature for a high-performance PPA and a fast-crystallizing grade. The fast-crystallizing grade sacrifices over 50º C (90º F) of performance. This is an excellent illustration of the continual tradeoff between processability and performance that we see in the world of plastics. If a change is made to a material that makes life easier for the processor, it almost certainly involves a reduction in field performance.
The converse is also true, and with most high-performance semi-crystalline materials, achieving the optimal structure requires the use of mold temperatures that cannot be achieved with traditional water heaters. Instead, pressurized water, oil, or heater cartridges are required. This can be a significant barrier to entry for some processors. Consequently, some processors attempt to anneal into the parts most of the crystallinity that is required. Instead of using the annealing process to produce the last 10% of the achievable crystallinity, they run the material at a low mold temperature that essentially results in an amorphous structure and then they “bake in” the crystal structure after the parts are molded.
While this may work in some cases, it is an approach fraught with downsides. These can be appreciated by illustrating what occurs structurally when a material that “wants” to be semi-crystalline is molded in an amorphous state and is then crystallized later. PEEK is a good example of a material that can be fabricated into an amorphous or a semi-crystalline structure, depending upon the rate at which the material is cooled. In fact, some data sheets for unfilled PEEK give a density for both the amorphous and the semi-crystalline form of the material. As a semi-crystalline material, the density is given as 1.30 g/cm3. In amorphous form it is 1.26 g/cm3.
Most of us know that semi-crystalline polymers shrink more out of the mold than amorphous materials. A typical mold shrinkage value for an unfilled amorphous polymer is 0.005 mm/mm or about 0.5%. Unfilled PEEK in semi-crystalline form shrinks approximately 1.5%. This may lead a processor seeking to “anneal in” the crystal structure of PEEK to calculate that the molded part dimensions will be reduced by approximately 1% during the annealing process. However, the difference in density between the amorphous and the semi-crystalline form suggests a volume change of over 3%. Why the discrepancy?
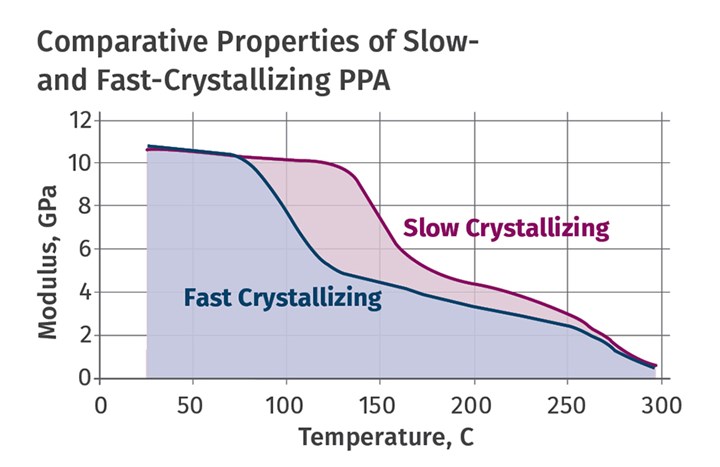
What many of us forget is that mold-shrinkage values are calculated from the way that parts shrink after they have been formed under significant pressure. As a material cools in a mold, we continue to apply pressure to compensate for the relatively large change in volume that would occur if the part were to cool in an unrestrained state. Amorphous polymers typically exhibit mold shrinkage values of approximately 0.5%, but we know that the melt density of an amorphous polymer is approximately 10% lower than that of the solid material. Without the constraints of the mold and the ability to apply pressure while the material is cooling, our experience of the way materials shrink would be very different.
Annealing takes place in an unrestrained environment. Therefore, the dimensional changes the part will exhibit during annealing will be much greater than expected from our experience as processors. In addition, a part molded at a relatively low mold temperature will contain a much higher degree of retained orientation in its structure. The material that forms the surface will have a substantially different structure than the material in the core, and during the annealing process the molded-in stress will tend to relax. This frequently results in warpage.
The dimensional changes that the part will exhibit during annealing will be much greater than expected from our experience as processors.
I once worked with a processor who had corrected a warpage problem in a long connector of a semi-crystalline material by reducing the mold temperature from 120 C (248 F) to 35 C (95 F). When the customer received the parts, it promptly placed them in an oven at 120 C and the parts warped to an even greater degree than they had coming out of the hot mold. The processor claimed that the customer was “cheating.” I explained to them that the customer was merely determining what would happen to the parts when they reached their operating temperature in the field and that the crystallinity that they had suppressed to correct the warpage problem would occur anyway once the part reached the operating temperature of the device.
The final problem with “annealing in” most or all of the crystalline structure goes back to something we mentioned in our last article: Crystals formed in the solid state through annealing are not as large or as perfect as crystals formed from the melt. It is known that crystals that are “annealed in” will melt at a temperature slightly higher than the temperature at which they were created. Consider PEEK again. A common annealing routine for PEEK is a 2-hr exposure to 200 C (392 F). This temperature is well above the Tg of the polymer and will effectively provide that last little bit of crystallinity that we could not obtain from the molding process.
But consider a part that has been molded in an essentially an amorphous state. When annealed at 200 C, assuming the part is still in a form that is recognizable, it will consist of crystals that melt at approximately 220 C (428 F). This is a long way from the typical melting point of 343 C (649 F) that we expect from the polymer. It is also below even the quoted continuous-use temperature for the material, which on the UL yellow card can be as high as 260 C (500 F).
Therefore, while it is technically possible to forgo the elevated mold temperatures normally associated with best practices for processing high-performance semi-crystalline materials, it is very risky and likely to yield parts that dramatically under perform expectations—assuming that they emerge from the annealing process looking anything like the drawing. In our next column we will extend the annealing discussion to its application in crosslinked materials.
ABOUT THE AUTHOR Mike Sepe is an independent, global materials and processing consultant whose company, Michael P. Sepe, LLC, is based in Sedona, Ariz. He has more than 40 years of experience in the plastics industry and assists clients with material selection, designing for manufacturability, process optimization, troubleshooting, and failure analysis. Contact: (928) 203-0408 • mike@thematerialanalyst.com.
Related Content
Prices for All Volume Resins Head Down at End of 2023
Flat-to-downward trajectory for at least this month.
Read MoreWhat's the Allowable Moisture Content in Nylons? It Depends: Part 2
Operating within guidelines from material suppliers can produce levels of polymer degradation. Get around it with better control over either the temperature of the melt or the barrel residence time.
Read MoreMelt Flow Rate Testing–Part 1
Though often criticized, MFR is a very good gauge of the relative average molecular weight of the polymer. Since molecular weight (MW) is the driving force behind performance in polymers, it turns out to be a very useful number.
Read MoreTracing the History of Polymeric Materials: Acetal
The road from discovery in the lab to commercial viability can be long, and this was certainly the case for acetal polymers.
Read MoreRead Next
Materials: Annealing Tips for Amorphous Polymers, Part 2
In amorphous polymers, annealing is performed to draw down the internal stresses to a level not achievable within the conditions of a normal molding process. But a few parameters are important to achieving the desired results.
Read MoreMaterials Part 1: What Annealing Can Do for Your Process
Relatively rapid cooling rates in processing introduce internal stress. If functional problems in use result, annealing may draw down the stress to levels that may not be achievable during processing.
Read MorePart 3 Materials: Annealing Tips for Semicrystalline Polymers
For these polymers, annealing is done to establish a level of crystallinity that cannot be practically obtained within the parameters of a normal molding cycle. Here’s some guidance on setting annealing time and temperature.
Read More